An efficient photo reactor design and its application in photo-oxidation of acetone
DOI:
https://doi.org/10.52493/j.cote.2023.1.57Keywords:
Acetone, photoreactor, photooxidation, photodecompositionAbstract
The rate of CO2 production obtained from the photodecomposition of acetone with different loadings of TiO2 as a photocatalyst is remarkable. This study aims to develop a flow-type photo reactor with excellent contact between all the reacting species. To achieve total mineralization with minimal waste, the flow rate of gases was controlled. During this investigation, the acetone was fed at the rate of 2.8 mmol h-1 and the air flow rate was maintained at 4.3 Lh-1. The reaction was carried out at near room temperature as the cold cathode lamps increased temperature very slowly to 63 ᵒC. No CO2 was produced either without light or catalyst indicating a photocatalytic reaction. There was a proportional increase in the production of CO2 with an increase in TiO2 loading. A maximum rate of 5.75 ± 0.07 mMh-1 for CO2 production was achieved with a catalyst loading of 5 mg/gbeads. Increasing the catalyst loading above 5 mg/g showed a decrease in CO2 production rate which can be attributed to reduced contact between light and catalyst. This technique used here can be adopted for the decomposition of various Volatile Organic Compounds (VOCs).
References
Coronado, J. M., Kataoka, S., Tejedor-Tejedor, I., & Anderson, M. A. (2003). Dynamic phenomena during the photocatalytic oxidation of ethanol and acetone over nanocrystalline TiO2: Simultaneous FTIR analysis of gas and surface species. Journal of Catalysis, 219(1), 219–230. https://doi.org/10.1016/S0021-9517(03)00199-4
De Lasa, H., Serrano, B., & Salaices, M. (2005). Photocatalytic reaction engineering. Photocatalytic Reaction Engineering. New York. https://doi.org/10.1007/0-387-27591-6
El-Maazawi, M., Finken, A. N., Nair, A. B., & Grassian, V. H. (2000). Adsorption and photocatalytic oxidation of acetone on TiO2: An in situ transmission FT-IR study. Journal of Catalysis, 191(1), 138–146. https://doi.org/10.1006/jcat.1999.2794
Fraters, B. D. (2015). TiO2 based photocatalytic gas purification; the effects of co-catalysts and process conditions. Gildeprint drukkerijen, Enschede, The Netherlands. https://doi.org/10.3990/1.9789036538862
Fujishima, A., Rao, T. N., & Tryk, D. A. (2000). Titanium dioxide photocatalysis. Journal of Photochemistry and Photobiology C: Photochemistry Reviews, 1(1), 1–21. https://doi.org/10.1016/S1389-5567(00)00002-2
Fujishima, A., Zhang, X., & Tryk, D. A. (2008). TiO2 photocatalysis and related surface phenomena. Surface Science Reports. https://doi.org/10.1016/j.surfrep.2008.10.001
Han, Z., Chang, V. W. C., Zhang, L., Tse, M. S., Tan, O. K., & Hildemann, L. M. (2012). Preparation of TiO2-Coated Polyester Fiber Filter by Spray-Coating and Its Photocatalytic Degradation of Gaseous Formaldehyde. Aerosol and Air Quality Research, 12(6), 1327–1335. https://doi.org/10.4209/aaqr.2012.05.0114
He, J., Zhai, Q., Zhang, Q., Deng, W., & Wang, Y. (2013). Active site and reaction mechanism for the epoxidation of propylene by oxygen over CuOx/SiO2 catalysts with and without Cs+ modification. Journal of Catalysis, 299, 53–66. https://doi.org/10.1016/j.jcat.2012.11.032
Henderson, M. A. (2008). Effect of coadsorbed water on the photodecomposition of acetone on TiO2 (110). Journal of Catalysis, 256, 287–292. https://doi.org/10.1016/j.jcat.2008.03.020
Horie, T., Sumino, M., Tanaka, T., Matsushita, Y., Ichimura, T., & Yoshida, J. I. (2010). Photodimerization of maleic anhydride in a microreactor without clogging. Organic Process Research and Development, 14(2), 405–410. https://doi.org/10.1021/op900306z
Krishnan, J., & Swaminathan, T. (2010). Kinetic modeling of a photocatalytic reactor designed for removal of gas-phase benzene: A study on limiting resistances using design of experiments. Latin American Applied Research, 40(4), 359–364.
Li, T., Zeng, W., Long, H., & Wang, Z. (2016). Nanosheet-assembled hierarchical SnO2 nanostructures for efficient gas-sensing applications. Sensors and Actuators, B: Chemical, 231, 120–128. https://doi.org/10.1016/j.snb.2016.03.003
Li, Y., Yu, H., Song, W., Li, G., Yi, B., & Shao, Z. (2011). A novel photoelectrochemical cell with self-organized TiO2 nanotubes as photoanodes for hydrogen generation. International Journal of Hydrogen Energy, 36(22), 14374–14380. https://doi.org/10.1016/j.ijhydene.2011.08.026
Lin, L., Chai, Y., Zhao, B., Wei, W., He, D., He, B., & Tang, Q. (2013). Photocatalytic oxidation for degradation of VOCs. Open Journal of Inorganic Chemistry, 03(01), 14–25. https://doi.org/10.4236/ojic.2013.31003
Liu, L., Zhao, H., Andino, J. M., & Li, Y. (2012). Photocatalytic CO2 Reduction with H2O on TiO 2 Nanocrystals: Comparison of Anatase, Rutile, and Brookite Polymorphs and Exploration of Surface Chemistry. ACS Catalysis, 2(8), 1817–1828. https://doi.org/10.1021/cs300273q
Nagda, N. L., & Rector, H. E. (2003). A critical review of reported air concentrations of organic compounds in aircraft cabins. Indoor Air, 13(3), 292–301. https://doi.org/10.1034/j.1600-0668.2003.00202.x
Nguyen, V.-H., Lin, S. D., Wu, J. C. S., & Bai, H. (2015). Influence of co-feeds additive on the photo-epoxidation of propylene over V–Ti/MCM-41 photocatalyst. Catalysis Today, 245, 186–191. https://doi.org/10.1016/j.cattod.2014.06.027
Raillard, C., Héquet, V., Le Cloirec, P., & Legrand, J. (2004). Kinetic study of ketones photocatalytic oxidation in gas phase using TiO 2 -containing paper: effect of water vapor. Journal of Photochemistry and Photobiology A: Chemistry, 163, 425–431. https://doi.org/10.1016/j.jphotochem.2004.01.014
Sakai, H., Kubota, Y., Yamaguchi, K., Fukuoka, H., & Inumaru, K. (2013). Photocatalytic decomposition of 2-propanol and acetone in air by nanocomposites of pre-formed TiO2particles and mesoporous silica. Journal of Porous Materials, 20(4), 693–699. https://doi.org/10.1007/s10934-012-9643-5
Song, H., Li, Y., Lou, Z., Xiao, M., Hu, L., Ye, Z., & Zhu, L. (2015). Synthesis of Fe-doped WO3 nanostructures with high visible-light-driven photocatalytic activities. Applied Catalysis B: Environmental, 166–167, 112–120. https://doi.org/10.1016/j.apcatb.2014.11.020
Verbruggen, S. W., Masschaele, K., Moortgat, E., Korany, T. E., Hauchecorne, B., Martens, J. A., & Lenaerts, S. (2012). Factors driving the activity of commercial titanium dioxide powders towards gas phase photocatalytic oxidation of acetaldehyde. Catalysis Science & Technology, 2(11), 2311-2318. https://doi.org/10.1039/c2cy20123b
Verbruggen, S. W., Ribbens, S., Tytgat, T., Hauchecorne, B., Smits, M., Meynen, V., ... & Lenaerts, S. (2011). The benefit of glass bead supports for efficient gas phase photocatalysis: case study of a commercial and a synthesised photocatalyst. Chemical engineering journal, 174(1), 318-325. https://doi.org/10.1016/j.cej.2011.09.038
Xu, W., & Raftery, D. (2001). In situ solid-state nuclear magnetic resonance studies of acetone photocatalytic oxidation on titanium oxide surfaces. Journal of Catalysis, 204(1), 110–117. https://doi.org/10.1006/jcat.2001.3380
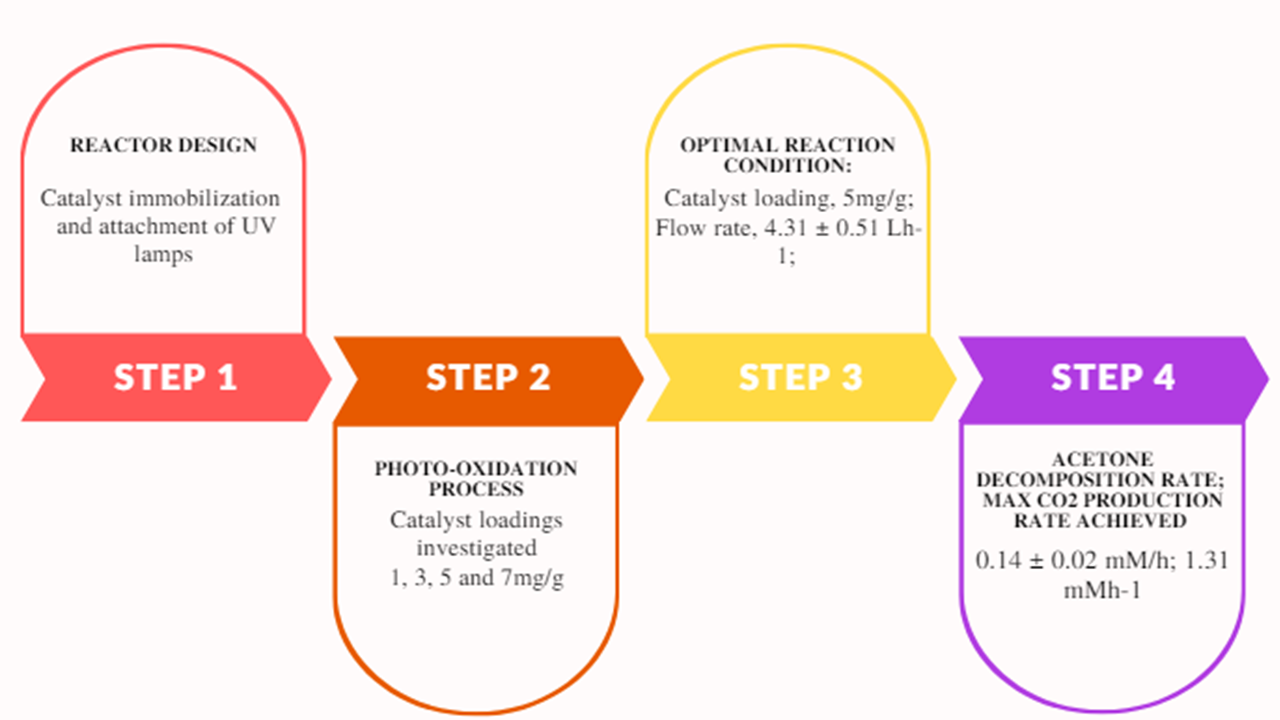
Downloads
Published
Issue
Section
License
Copyright (c) 2023 Emmanuel A. Kamba, Daniel I. Ugwuja, Emmanuel A. Yerima, Godwin O. Egah

This work is licensed under a Creative Commons Attribution 4.0 International License.